The age of expendable launch vehicles
Until recently, the future of space launch looked pretty much like its past. For over 50 years, humanity has blasted thousands of spacecraft and satellites into space. Every launch vehicle that carried them aloft has ended up littering the planet with the broken, twisted remains of expended rocket stages. The scenario goes something like this:
- A multi-stage rocket blasts off from its launch site.
- After a few minutes, the largest, most expensive stage (known as the first or booster stage) shuts down and separates from the rest of the vehicle.
- This discarded booster stage then falls back to Earth, crashing in an ocean or some bleak, uninhabited wasteland.
- The rest of the launch vehicle continues on, throwing away stages, each one smaller and less powerful than the last.
- The final stage deploys the payload at its targeted trajectory and velocity.
- Eventually, even this final stage burns up in the atmosphere when its orbit decays after months or even years in space.
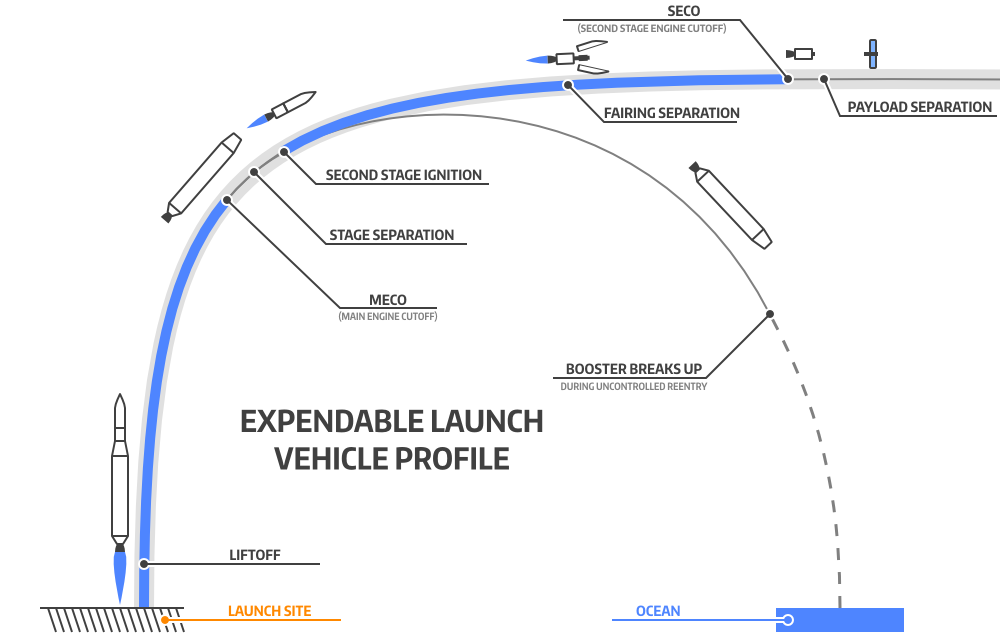
Only a tiny fraction of the entire rocket escapes Earth’s deep gravity well to reach space as useful payload. With one notable exception, the Space Shuttle, it seemed like a virtual impossibility to recover any part of the launch vehicle. Because of this assumption, expendable launch vehicles were deemed to be just ‘the cost of doing business’… until now.
A new way of doing business
This wasteful and costly method of getting payloads to space could be about to change in the near future. The California-based company Space Exploration Technologies, or SpaceX, has finally revealed how and when they will attempt to recover the booster stage of its Falcon 9 launch vehicle.
There were many clues that SpaceX would make this bold attempt for some time. SpaceX’s founder and chief designer, Elon Musk, has stated that rapid recovery and reuse of the Falcon 9 booster stages was a priority for his company. His favorite analogy is that nobody would fly in disposable airplanes because discarding them after every flight would make air travel prohibitively expensive. But, like the pioneering days of aviation a century ago, an incremental approach had to be followed to achieve the goal of fully reusable booster stages.
First, there was the Grasshopper test vehicle constructed from Falcon 9 booster stage tanks, a single Merlin rocket engine, and fixed steel legs. It made a number of increasingly complex vertical takeoff and landing flights over SpaceX’s test facility in central Texas. Those short hops proved that the Grasshopper’s rocket engine could be controlled with enough precision to make a safe, on-target landing.
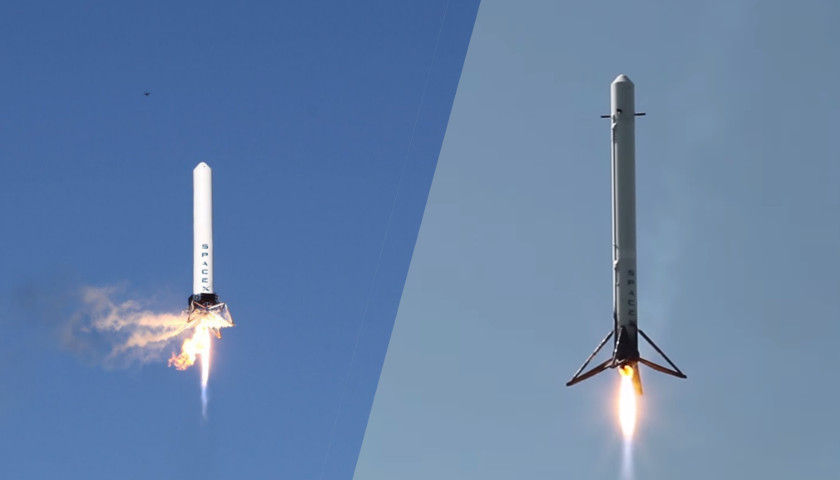
Next came a follow-up test vehicle called the Falcon 9R-Dev1, the ‘R’ in the name standing for ‘reusable’. Like the Grasshopper before it, this new vehicle was constructed with the same tanks and rocket engines used on the Falcon 9 booster stages. Instead of the Grasshopper’s steel legs, its carbon composite landing legs would be folded against the side of the booster stage for launch and deployed before landing. After only a few successful flights, the vehicle self-destructed when an engine failure sent it off-course; thus showing, yet again, just how hard rocket science can be.
As these test flights were occurring in Texas, SpaceX was attempting to land booster stages in the ocean during actual space launches. This would demonstrate that it was possible to safely land on solid ground during future flights. The first attempt was during the inaugural flight of the newly upgraded Falcon 9, version ‘1.1’ of SpaceX’s launch vehicle. It was also the first use of their new launch site at Vandenberg Air Force Base in California. After sending its upper stage and payload onward to orbit, the booster stage fired three of its nine engines to slow its velocity enough for a safe reentry into the atmosphere. Although it survived, the single engine it used during the landing phase shut down prematurely for lack of fuel due to an uncontrollable roll. The unpowered booster stage crashed into the Pacific Ocean at high speed.
Undaunted, SpaceX made two more tries to land on water during launches for paying customers from their original launch site at Cape Canaveral. The first flight was a Dragon capsule cargo mission to the International Space Station (CRS-3). The other flight launched six small, low orbit communication satellites for a company called Orbcomm (OG2 Mission 1). For the first time, the new landing legs were installed on both booster stages. SpaceX had stated that the boosters were unlikely to survive after their engines shut down and they tipped over. Video and telemetry data from both landing attempts showed that they had touched down softly in the Atlantic Ocean. As SpaceX predicted, neither booster stage was recovered; despite this, the two attempts were considered successes as they had shown complete control during the reentry and landing phases of the flights.
So it’s not as if we, the general public, didn’t know that SpaceX fully intended to recover and reuse Falcon 9 booster stages. Still, there were a few pieces of the puzzle missing before they could make this a reality. SpaceX had said that it would try more ocean landings closer to shore as an incremental step toward finally landing near their launch site (which would facilitate the quicker reuse of the booster stages). There was even talk about landing on an ocean-going platform that was being constructed, but few details about this vessel were made available.
Sometimes, all you have to do is ask
On Saturday, November 22nd, Elon Musk posted an image on Twitter. “Testing operation of hypersonic grid fins (x-wing config),” he said. “[Going] on [the] next flight.”
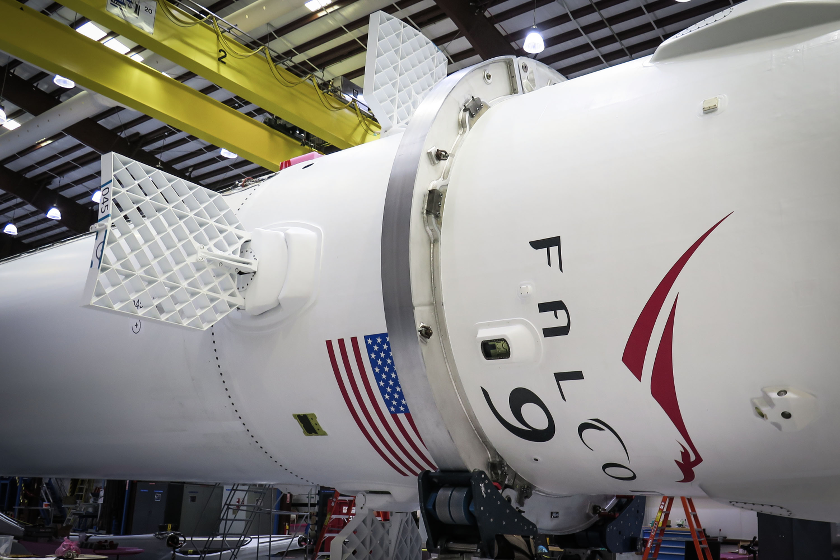
The image shows a Falcon 9 launch vehicle in its hangar with four ‘grid fins’ sticking out of the side of the vehicle. These were similar to ones that had already been successfully tested on the Falcon 9R-Dev1 before its ill-fated last flight. The new fins are designed to improve control of the booster stage on its way down to a precision landing. According to Elon Musk, the fins will debut on the next cargo mission to the International Space Station (CRS-5). Even though he had announced that the new ocean-going landing platform might be used on this flight as well, his followers wanted more. @John_Gardi asked him to reveal this vessel to the public:
@elonmusk: You
go guy! How about a picture of the landing barge! THAT
would cause a stir (& put the fear into booster makers
everywhere!).
— Just A. Tinker (@John_Gardi)
November
22, 2014
Much to everybody’s surprise, Elon Musk replied almost immediately:
Autonomous spaceport drone ship. Thrusters repurposed
from deep sea oil rigs hold position within 3m even in a
storm. pic.twitter.com/wJFOnGdt9w
— Elon Musk (@elonmusk)
November
22, 2014
The ‘drone ship’, as Elon Musk calls it, seems to be an ocean-going barge with a landing platform the size of a football field constructed on top. It would remain stationary at a precise location using four diesel-powered hydraulic underwater thrusters mounted to the corners of the barge. All indications are that SpaceX intends to recover at least the first few booster stages by using this drone ship as a landing pad.
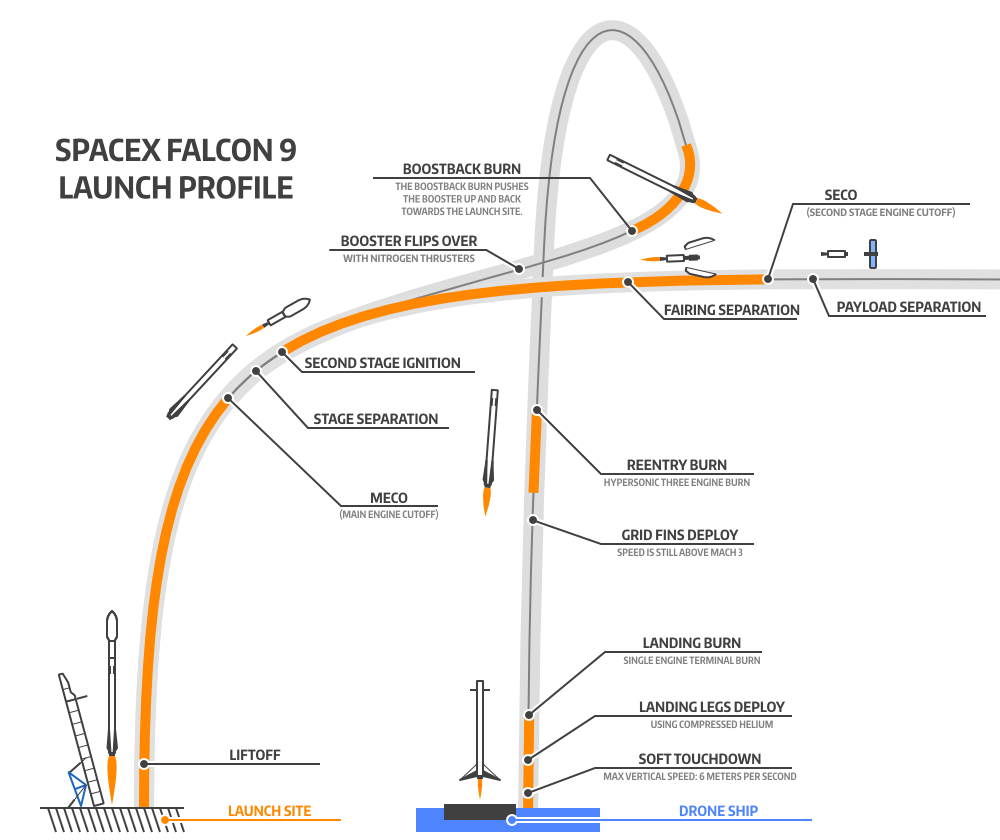
Elon Musk has said that if the first attempt to land a booster stage on the drone ship fails, there’ll be many launches afterwards to try again. SpaceX’s successes so far have made this look like an easy task. However, many have already attempted to follow the path toward reusable launch vehicles… and failed.
The rocky road to reusable launch vehicles
The booster stage is the largest and most expensive single component of a launch vehicle. Even though the booster’s job is done in just a few short minutes, it does the majority of the work needed to get a payload into space. Without these booster stages, space launch would be impossible.
Engineers don’t design launch vehicles to run for a short period of time and then fall apart; they build them to the highest standards to endure the extreme forces and vibrations associated with space launch. Rocket engines are tested far beyond their few minutes of necessary survival, often running for dozens of flights’ worth of time on a test stand to ensure their reliability.
Any recovery system adds extra weight and cost to the entire launch vehicle. This cuts into the already small amount of weight allocation meant for the payload, which is the whole purpose of space launch in the first place. If engineers tried to add a recovery system to an existing launch vehicle, its former payloads would be too heavy to be launched on that vehicle at all. The fact that recovering and reusing the booster stage would greatly lower the cost of space launch is lost on most launch vehicle manufacturers. Their thinking seems to be that if the recovery system takes away half of the weight allocated to the payload, the cost by weight to the customer would be doubled. Since no other alternatives are available, the use of expendable launch vehicles has almost become a tradition.
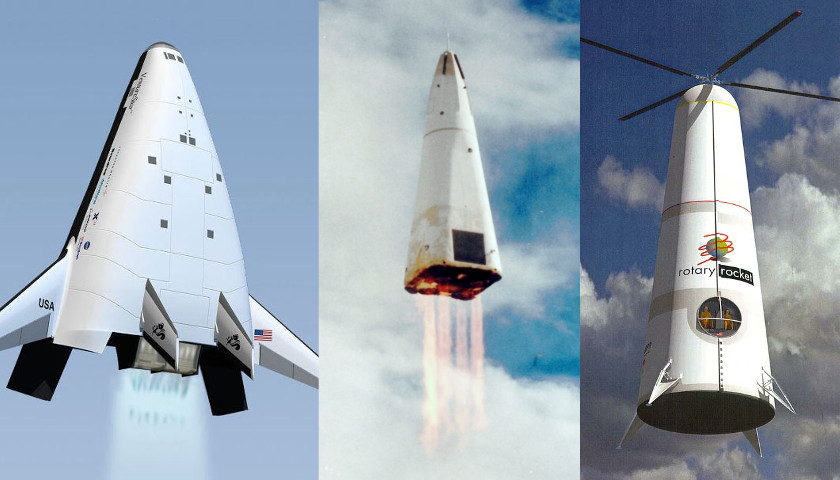
Reusable launch vehicles have been attempted before; most failed to get beyond the design phase. Among the few prototypes that actually got built were the Lockheed Martin VentureStar and the McDonnell Douglas DC-X. The VentureStar concept was scrapped when the prototype’s fuel tanks exhibited major structural flaws. The DC-X test program was transferred to NASA after a number of successful test flights. It was then canceled due to budgetary and management concerns. In the late ’90s, an American company called Rotary Rocket got as far as building and testing a full-scale ‘Atmospheric Test Vehicle’ of their Roton ‘Single Stage To Orbit’ (SSTO) launch vehicle. Soon afterwards, they went bankrupt for lack of investor interest.
But one vehicle claiming to be reusable actually did get completed and utilized for three decades: NASA’s Space Transportation System, or the Space Shuttle, as we’ve come to know it.
As it was originally conceived by NASA, the Space Transportation System (STS) was intended to be a completely reusable bridge to space. It was meant to be refurbished and relaunched in days, each vehicle in the fleet flying dozens of times a year. That frequent launch rate alone would have reduced the cost of getting payloads into orbit by a substantial amount. But the final design, modified to accommodate Air Force requirements, ended up being only a partially reusable launch system. It took months, not days, to refurbish at a cost per launch of half a billion dollars; the same cost as a single Saturn V moon rocket launch.
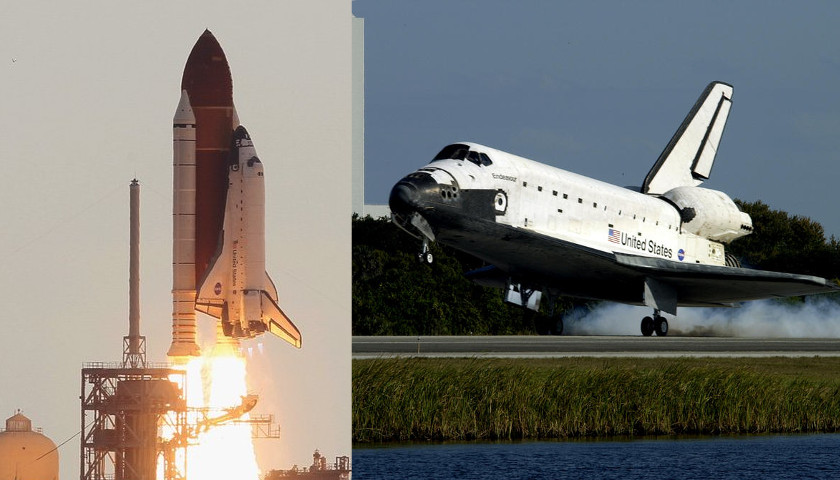
The Space Transportation System’s assembly started with two solid fuel rocket boosters, the largest ever constructed at the time. Each booster was made up of four sections stacked atop of each other; the first section was bolted to the launch platform and supported the weight of the entire vehicle. Between them was mounted a fifteen story tall tank that held liquid hydrogen and oxygen, used to feed the Space Shuttle Orbiter’s three rocket engines. This Orbiter was a delta winged, crew carrying space plane with a large payload bay in its fuselage; during launch, it was mounted to the side of the External Tank. The flight profile of this ungainly vehicle went as follows:
- The Orbiter’s three engines ignite at T-6 seconds; health tests are performed while they throttle to full power.
- The twin solid rocket boosters ignite at T-0 and explosive bolts on the launch platform release the vehicle.
- The solid rocket boosters burn for a minute and a half before they run out of fuel; explosive bolts jettison them from the External Tank.
- After a ballistic reentry into the atmosphere, parachutes lower the expended solid rocket boosters into the ocean; they are later towed back to shore.
- The Orbiter, with the external tank still mounted to its underside, continues on until its fuel and oxidizer are almost used up.
- Before the vehicle reaches orbit, the External Tank is jettisoned; it’s destroyed during reentry.
- The Orbiter then fires its two rear mounted Orbital Maneuvering System engines to give it the final push needed to achieve orbit.
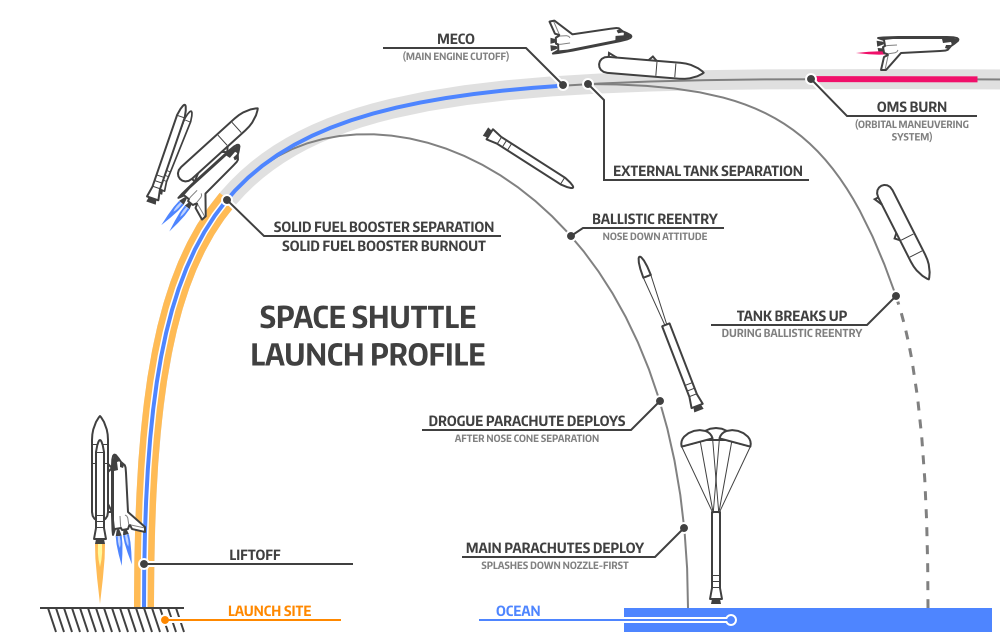
Even though the Space Shuttle was the most versatile spacecraft ever built, as an affordable and reusable launch system, it was an utter failure. In fact, the Space Shuttle has been used as an example to argue that affordable, reusable launch vehicles themselves were an unfeasible dream.
It seemed like the hammer was raised to pound the last nail into the coffin of launch vehicle reusability the day the final Space Shuttle mission landed.
The stairway to heaven
Elon Musk has bucked the decades-old paradigm of launch vehicle expendability since the day he founded SpaceX in 2002. He hired uniquely experienced engineers such as Tom Mueller and Gwynne Shotwell. Mueller was a rocket engine designer for the aerospace company TRW. Under his leadership, his team developed and built a number of low-cost rocket engines, including a direct ancestor of the Merlin engine SpaceX uses today. Shotwell had worked in the aerospace industry for fifteen years in various fields before joining SpaceX. Her wide-ranging skills earned her the position of President and COO of the company. Part of her job was to attract customers to risk their expensive payloads on a new, unproven launch vehicle. Other highly skilled engineers, young and old, were attracted to SpaceX by the prospect of designing and building the reusable launch vehicles of the future.
Elon Musk and his SpaceX team threw out the ‘Rocket Builder’s Handbook of the Twentieth Century’ and started from scratch with their own designs.
- Instead of using a foundation of flight-tested but badly outdated designs, they designed a brand-new rocket, built using modern materials and techniques.
- Instead of building high-performance rocket engines with exotic alloys to squeeze out every ounce of performance, they sacrificed some performance for a large reduction in cost.
- Instead of building a larger, more powerful rocket engine for the booster stage, they designed it to use nine smaller ones.
- Instead of using expensive radiation-hardened computers, they chose a low-cost design using three redundant computers built from ‘off the shelf’ components.
- Instead of using flight computers in the uppermost stage alone, they used identical avionics in both stages to facilitate booster stage recovery.
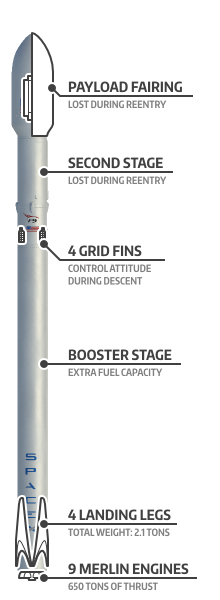
Not only did using nine booster engines provide assurance that a launch would succeed even if an engine failed, it opened up options to recover the booster stages at some later date. By using virtually identical engines on both the booster and the second stage, they would also get more engine performance data per launch while reducing both cost and design complexity.
SpaceX also designed the booster stage of the Falcon 9 to have far more power than was required to launch the most common medium-weight payloads into space. This extra power provided the margin SpaceX needed to develop a recovery system without having to take a future hit on the vehicle’s payload capacity. Even as an expendable launch vehicle, the Falcon 9 is already one of the lowest-cost in its class, putting the company ahead of its competition even without reusability.
The recovery system that SpaceX finally arrived at was nothing more complicated than fuel, landing legs, grid fins, and updated flight software. This system took advantage of the vehicle’s extra fuel capacity and lighter weight to create a reusable booster stage out of an expendable one. Reusability could then be achieved with minimal structural changes to the existing booster stage.
So now SpaceX finally has all the pieces in place to actually recover the booster stage of a Falcon 9 launch vehicle for the very first time. Those booster stages were designed from the beginning to be reused and SpaceX’s ‘test while you fly’ approach is about to pay off. The implications of this are profound. Recovering the most expensive part of the launch vehicle for reuse would reduce the cost of space launch to a fraction of the going rate. With low-cost, rapidly reusable booster stages, SpaceX will be able to launch payloads into space for much smaller commercial companies, universities, and new enterprises. One such idea is the micro-satellite constellation hinted at by Elon Musk himself, which could bring low-cost internet access to the most remote locations on Earth.
Elon Musk and SpaceX are leading the way through to a new frontier, preparing to lay down a road to humanity’s future amongst the stars.